Zeeman Effect vs. Stark Effect: What's the Difference?

Edited by Janet White || By Harlon Moss || Updated on October 16, 2023
The "Zeeman effect" relates to splitting of atomic spectral lines due to an external magnetic field, while the "Stark effect" involves spectral line splitting due to an external electric field.

Key Differences
The "Zeeman effect" and "Stark effect" both pertain to the splitting of spectral lines in atomic physics, but they arise from distinct external influences. The "Zeeman effect" manifests when an atom is exposed to an external magnetic field, causing the energy levels of the atom to shift and the spectral lines to split.
Contrarily, the "Stark effect" emerges in response to an external electric field. Here, the energy levels of the atom also experience a shift, leading to a discernible splitting of the spectral lines, similar to the "Zeeman effect", but due to a different mechanism.
It's crucial to note that while both effects illustrate the perturbation of atomic energy states, the fundamental force at play varies. In the "Zeeman effect", it's the interaction of the atom's magnetic moment with the external magnetic field, whereas in the "Stark effect", it's the atom's electric dipole moment interacting with the external electric field.
At a deeper quantum mechanical level, the "Zeeman effect" can further be categorized into normal and anomalous types, based on electron spin interactions. In comparison, the "Stark effect" doesn't inherently deal with such categorizations but focuses primarily on electric field-induced perturbations.
To sum it up, while both the "Zeeman effect" and "Stark effect" revolve around the phenomenon of spectral line splitting, they are dictated by distinct external fields - magnetic for the former and electric for the latter.
ADVERTISEMENT
Comparison Chart
External Field
Magnetic field
Electric field
Cause
Interaction of atomic magnetic moment with the magnetic field.
Interaction of atomic electric dipole moment with the electric field.
Observed in
Atoms with magnetic moments.
Atoms with electric dipole moments.
Quantum Mechanics Classification
Can be normal or anomalous based on electron spin.
Primarily electric field-induced perturbations.
Application
Magnetic field strength measurement.
Electric field strength measurement.
ADVERTISEMENT
Zeeman Effect and Stark Effect Definitions
Zeeman Effect
The splitting of spectral lines due to an external magnetic field.
Studying the Zeeman effect helps understand atomic magnetic moments.
Stark Effect
A representation of electric field-induced perturbations in atomic energy levels.
Quantum mechanics was enriched by the study of the Stark effect.
Zeeman Effect
Magnetic resonance of atomic spectral lines.
He calibrated the instrument using the Zeeman effect.
Stark Effect
A method to analyze atomic electric properties through spectral examination.
The Stark effect was utilized to probe the atomic structure.
Zeeman Effect
A tool for probing atomic magnetic properties via spectral lines.
The detection of the Zeeman effect confirmed the presence of a magnetic field.
Stark Effect
The splitting of atomic spectral lines due to an external electric field.
The Stark effect provides insight into atomic electric dipole moments.
Zeeman Effect
A manifestation of magnetic field interactions in atomic physics.
By observing the Zeeman effect, they gauged the magnetic field's strength.
Stark Effect
Electric resonance observed in atomic spectral lines.
Using the Stark effect, she measured the strength of the electric field.
Zeeman Effect
A phenomenon illustrating magnetic field-induced atomic energy level perturbations.
The Zeeman effect was critical in the development of quantum mechanics.
Stark Effect
A phenomenon showing electric field interactions with atoms.
Evidence of the Stark effect confirmed the electric field's influence.
FAQs
Why is the Zeeman Effect observed?
It's observed due to the interaction of the magnetic moment of an atom's electron with an external magnetic field.
What is the Zeeman Effect?
The Zeeman Effect refers to the splitting of a spectral line into multiple components in the presence of a magnetic field.
Who discovered the Zeeman Effect?
The Zeeman Effect was discovered by the Dutch physicist Pieter Zeeman in 1896.
What causes the Anomalous Zeeman Effect?
It's caused by the combined effect of the orbital and spin magnetic moments of the electron.
How many types of Zeeman Effect are there?
the Normal Zeeman Effect, the Anomalous Zeeman Effect, and the Paschen-Back Effect.
What is the Stark Effect?
The Stark Effect refers to the splitting of a spectral line due to an external electric field.
How is the Zeeman Effect related to electron transitions?
The splitting of spectral lines indicates transitions between different magnetic energy levels of an atom in a magnetic field.
Why is the Stark Effect observed?
It's due to the interaction of the electric dipole moment of an atom or molecule with an external electric field.
How does the Stark Effect differ from the Zeeman Effect?
While the Zeeman Effect deals with magnetic fields, the Stark Effect is concerned with electric fields.
Is there a quantum mechanical basis for the Stark Effect?
Yes, the effect can be explained using quantum mechanics, particularly the perturbation theory.
Does the Stark Effect affect all atoms and molecules?
The effect is most pronounced for polar molecules with a permanent electric dipole moment but can also be observed in non-polar molecules under strong electric fields.
How does the Stark Effect contribute to atomic clocks?
The Stark Effect can cause frequency shifts in atomic clocks, so understanding and compensating for it is essential for precision timekeeping.
How is the Zeeman Effect used?
It's used in experimental setups to measure the magnetic fields of celestial bodies and in atomic absorption spectrometry.
Who discovered the Stark Effect?
The Stark Effect was discovered by the German physicist Johannes Stark in 1913.
Can the Stark Effect be used to measure electric fields?
Yes, it's a valuable tool for measuring internal and external electric fields at atomic and molecular levels.
Can the Zeeman Effect be observed for all spectral lines?
No, only spectral lines associated with appropriate magnetic quantum number changes will display the effect.
Is the Stark Effect important in astrophysics?
Yes, it helps in understanding the electric fields in various celestial environments and in the analysis of radiation from stars.
What are linear and quadratic Stark effects?
Linear Stark effect refers to the first-order perturbation due to the electric field, resulting in linear energy shifts. The quadratic Stark effect involves second-order perturbations, leading to quadratic energy shifts.
About Author
Written by
Harlon MossHarlon is a seasoned quality moderator and accomplished content writer for Difference Wiki. An alumnus of the prestigious University of California, he earned his degree in Computer Science. Leveraging his academic background, Harlon brings a meticulous and informed perspective to his work, ensuring content accuracy and excellence.
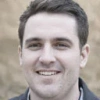
Edited by
Janet WhiteJanet White has been an esteemed writer and blogger for Difference Wiki. Holding a Master's degree in Science and Medical Journalism from the prestigious Boston University, she has consistently demonstrated her expertise and passion for her field. When she's not immersed in her work, Janet relishes her time exercising, delving into a good book, and cherishing moments with friends and family.
